Lucy Main
MPhys Physics student, University of Oxford
Email: [email protected]
In the UK, domestic buildings account for around a third of the energy budget. One fifth were built pre-1919, giving us some of the oldest housing stock in the world. Accordingly, retrofitting this stock, which forms 80% of the homes needed for 2050, is one of the most cost-effective ways to reduce energy consumption. Modelling suggests that using efficiency measures to achieve a 25% reduction in energy use by 2035 would save households an annual average of £270 and create over 60 thousand new jobs. There would also be tangible benefits to occupant health: the NHS could save an estimated 42p for every £1 spent on retrofitting fuel poor homes.
But what constitutes a net zero energy building (NZEB)? Since 2021, an EU directive has required all new buildings to be ‘nearly zero energy’. Renewable energy should ‘to a very significant extent’ cover the ‘very low’ energy demand; in practice, the performance threshold is determined by Member States, accounting for their specific climate and housing stock. The US Department of Energy stipulates that an NZEB’s ‘actual annual delivered energy’, on a ‘source’ basis, must not exceed ‘on-site renewable exported energy’. However, many publications use an ‘on-site’ basis, simplifying calculations by ignoring the generation mix and supply chain consumption.
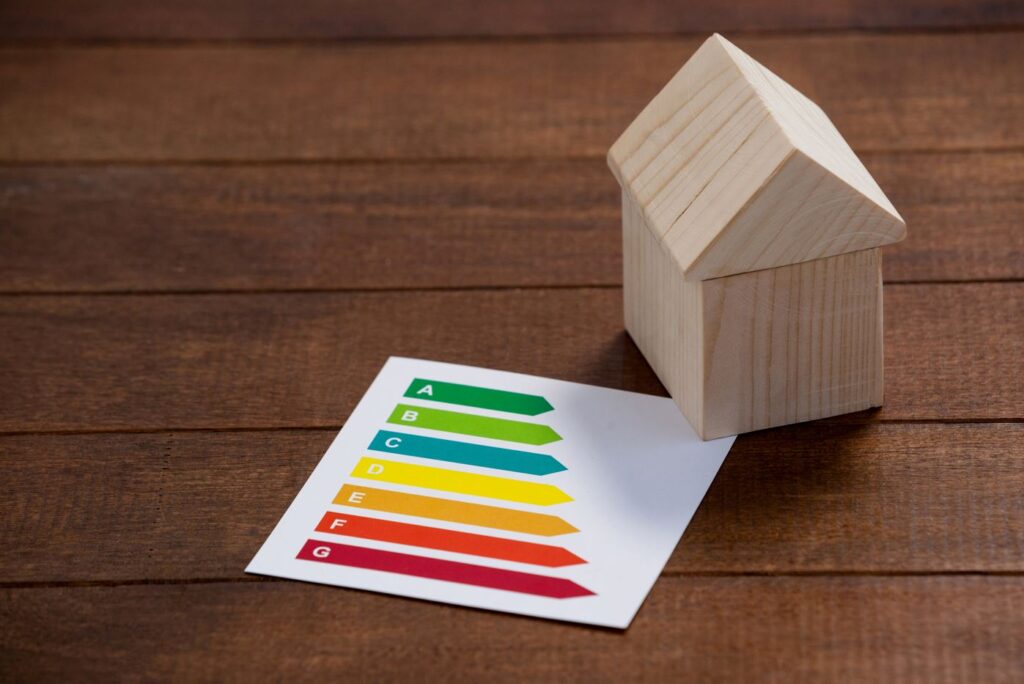
Many energy saving strategies are passive. The passive house (PH) standard, most applicable to colder climates where space heating dominates consumption, targets 15 kWh/m² annual heating load intensity. This is achieved with improved insulation and reduction in thermal bridging, eliminating the need for conventional heating. Airtightness demands mechanical ventilation with heat recovery to retain heat while providing fresh air. Retrofitting buildings to this standard often necessitates high up-front costs, although they may pay for themselves in the long-term, and can risk occupant health and structural integrity when significant building envelope changes create unforeseen moisture and ventilation problems.
Retrofits seldom reach new-build standards, as existing characteristics (shape, orientation, heritage status, neighbouring structures) can limit options. Nevertheless, successful PH retrofits exist of pre-1900 cottages and Brooklyn brownstones. A case study of fifty Canadian homes found that deep envelope retrofits and heat pumps could reduce community energy consumption by 69%, with net-negative homes compensated for by net-exporting neighbours. However, retrofitting requires extensive analysis to choose bespoke, cost-effective measures and can suffer when tradespeople lack knowledge and resources. Storage technology is currently insufficient to make off-grid NZEBs realistic, but future PHs with renewable resources may be able to store energy in times of excess to meet future shortfalls, or even enable homeowners to sell to the grid when demand is high.
In climates where cooling dictates energy use, passive design instead emphasises building shape, shading, window-to-wall ratio, and site layout. These considerations are vital, as solar radiation can increase energy consumption by up to a quarter. The best strategies vary between locations, but a study found that typical annual energy use could be halved in the Middle East and North Africa region. Photovoltaic (PV) systems are increasingly competitive in hot climates and provide a sustainable way to compensate for cooling demands, although, in areas with higher population densities and more multi-residential buildings, there is inevitably less space for PVs and natural light is restricted by neighbouring structures. However, a ‘double-skin green façade’ of indigenous plants on multi-storey dwellings could reduce energy consumption by three quarters, whilst improving humidity, air quality, biodiversity, noise insulation and reducing CO2 levels.
Vernacular architecture can also provide inspiration: traditional passive measures were modelled to reduce the annual energy demand of an average Mauritian house from 24 to 14 kWh/m². Similar strategies in Cuban architecture – wind permeable fibres, shade from foliage and pitched rooves for diffuse daylight, rainwater collection and convective ventilation – provide comfort when both temperature and humidity are high. Adobe (clay/mud) was once widely used as a construction material in Saudi Arabia. It is durable, locally available, inexpensive and less carbon intensive than reinforced concrete. One model suggested that it could reduce annual electricity use by 6%, whilst mushrabiyah (Islamic oriel windows providing natural ventilation and diffused natural light) resulted in a 4% reduction.
Controlling for the many variables that affect household performance is a major challenge to NZEB construction. Simulations indicate that energy consumption can vary by more than 10% with weather. Moreover, anthropogenic climate change means measures must be robust enough to provide enough energy and maintain efficiency under more extreme weather conditions and at higher average temperatures. This is particularly relevant to semi-arid regions, where cooling demand could increase by over 10% by the end of the century.
Occupant behaviour is crucial. Energy consumption can increase by over 100% with wasteful behaviour, potentially undermining gains made elsewhere. Occupants need information to get the most out of high-efficiency systems, particularly where smart technologies demand familiarity with user interfaces or necessitate certain behavioural changes. Half of surveyed residents in highly insulated Norwegian houses reported that bedroom temperatures were too high, with many opening windows to improve comfort at night. By creating a uniform temperature profile, the PH design inadvertently encouraged behaviour that compromised its airtightness. Fortunately, a review of energy consumption in over 2000 newly built PHs found that they generally suffered a smaller ‘performance gap’ than non-PH houses, probably due to the wide variation in occupants’ space heating behaviours. Overall, they achieved the PH standard with an average annual space heat energy consumption of 14.6 kWh/m².
A 2009 study identified seventeen actions (ranging from retrofitting to energy efficient driving) and used empirical studies to account for ‘plasticity’ – the proportion of the population who could be induced to change their behaviour. It demonstrated that 20% of US direct household emissions could be saved after a decade by national implementation of these actions, more than the total emissions of France. This is testament to the active role occupants can play in saving household energy in their everyday lives.